March 24, 2025 feature
This article has been reviewed according to Science X's editorial process and policies. Editors have highlighted the following attributes while ensuring the content's credibility:
fact-checked
peer-reviewed publication
trusted source
proofread
Might the proton decay in other places or at other times?
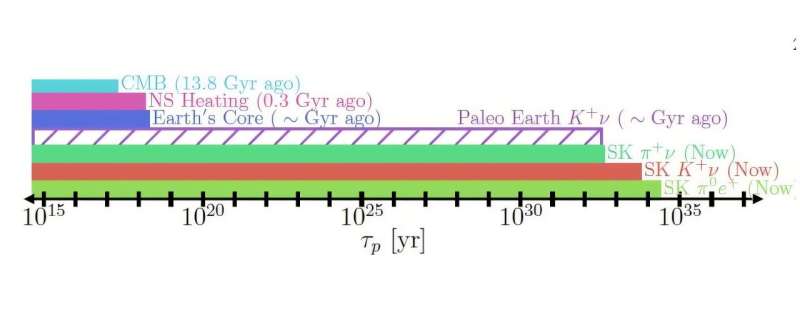
Does the proton decay? While this was a famous prediction of Grand Unified Theories (GUTS) developed in the 1970s and 1980s, experimentalists have ruled it out—or rather, put lower limits on its mean lifetime of about 1034 years. That's 24 orders of magnitude greater than the age of the universe.
But two physicists have been wondering: Could the lifetime be different in other places and at other times? Could the proton have decayed faster in the past? Could it decay faster somewhere else in the universe? They have reimagined some physics processes assuming the proton does decay and calculated possible lifetimes of around 1018 years. That's only eight orders of magnitude beyond the universe's lifetime. Their work was recently published in Physical Review D.
"People had previously asked various questions of the type, 'Are the fundamental physics parameters measured on the Earth the same elsewhere in the universe?'" said Peter Denton, a co-author at Brookhaven National Laboratory on Long Island, New York in the U.S. "One case that hadn't been investigated was the stability of the proton. Earth experiments show that the proton is incredibly stable, but those only apply here, in our part of the galaxy, and now, over the last several decades. What if proton decay depended on time or space?"
To flesh out this possibility, Denton and Hooman Davoudiasl, also at Brookhaven National Laboratory, considered possibilities where the heat from proton decay would show up in the development of the Earth's iron core, and in a neutron star. In GUTs, proton decay could proceed by many channels involving neutral or charged pions, electrons or muons, neutrinos, or kaons in different combinations, all of which have different theoretical lifetimes.
Here the authors considered a decay channel of a proton decaying into a positive pion and a dark fermion, "dark" here meaning it doesn't interact with regular stuff very much—the particles in the Standard Model that experience the electromagnetic interaction. If the dark particle's mass is less than the proton mass minus the pion mass, then the proton decay could be much faster than laboratory experiments imply, such as the limit from the Super-Kamiokande neutrino detector in Japan.
They first considered the Earth's solid iron core. If protons decay as above, producing heat, what lifetime is implied by the fact that the inner core isn't now molten? Because the inner core wasn't always solid—geologists believe it's been solid for about 1 to 1.5 billion years; other estimates range from 0.7 to as high as 4.2 billion years. The authors find that heat production of about four times that from the current inner core, which radiates about 1013 watts as a blackbody at 6,200 K, would melt the solid ball. Since it isn't molten, they find the proton lifetime must be greater than 2 × 1018 years.
Looking again for anomalous heat production, they considered a neutron star. The coldest known pulsar PSR J2144–3933 is known, from the Hubble Space Telescope, to have a temperature less than 42,000 K, and is known to be about 0.3 billion years old. (All pulsars are neutron stars, but not all neutron stars are pulsars).
Assuming a mass of about 1.4 solar masses, and knowing that protons are about 10% of a neutron star's baryonic content, this neutron star contains about 2 × 1056 protons. Putting their calculations together, Davoudiasl and Denton find that the proton lifetime must be greater than 1.5 x 1018, encouragingly close to the estimate from the inner core.
Constraints on the decay products of (the usual) dark matter and constraints from the Cosmic Microwave Background gave another lifetime lower limit of 2 × 1017 years. They also considered several "paleo detector" scenarios, such those outlined by Baum et al: protons decaying into an antineutrino and a K+ (K is for Kaon, a heavier cousin of the pion.)
This experiment couldn't be conducted on Earth because of its large atmospheric neutrino-induced backgrounds, but it might someday be conducted on the mineral olivine extracted from the moon a few kilometers below the surface. This measurement would provide a constraint on the total number of protons that have decayed over the last billion years.
Another constraint comes from examining the very low ratio (2 × 10-18) of 14C/12C in natural gas. Proton decay would be an additional source of 14C, giving a lower limit of about 1019 years.
Davoudiasl said examination of proton decay far from Earth or at much earlier times "could be realized, for example, if a hypothetical long-range force can affect the mass of a new particle into which a proton can decay, making the proton lifetime much shorter when those decays are energetically allowed."
There is an abundance of sources for this hypothesized force, which can change near the solar system as the sun orbits the Milky Way center every 230 million years, which would lead to a proton lifetime that would vary with space and time.
More information: Hooman Davoudiasl et al, How fast can protons decay? Physical Review D (2025). DOI: 10.1103/PhysRevD.111.035026
Journal information: Physical Review D
© 2025 Science X Network